Synthesis and in vitro bioactivity of sodium metasilicate-derived silicon-substituted hydroxyapatite
Keywords:
Silicon-substituted hydroxyapatite, Bioactivity, Sodium metasilicate, Bone repair, Synthetic bone graftsAbstract
Structural alteration of synthetic implants aims to achieve better bioactivity, higher cellular response, and regulated degradability, all of which are critical criteria for a biomaterial to serve as a graft in bone regeneration. The aim of this work was to synthesize silicon-substituted hydroxyapatite and test its bioactivity in simulated body fluid (SBF) by proving the use of sodium metasilicate (Na2SiO3.9H2O) as an affordable precursor of silica. Thus, the study evaluated the in vitro bone-bonding capacity of hydroxyapatite (Ca10(PO4)6(OH)2) (HA) substituted with silicate ion (Ca10(PO4)6-x(SiO4)x(OH)2-x; SixHA). The SixHA with x = 0.4 was synthesized by utilizing a wet precipitation method with sodium metasilicate as a low-cost silica alternative for alkoxysilane precursors. The SixHA was then examined for properties such as morphology, elemental composition, phase composition, and the nature of chemical bonds using scanning electron microscopy (SEM), energy dispersive X-ray analysis (EDX), X-ray diffractometry (XRD), and Fourier transformed infrared spectroscopy (FTIR), respectively. An in vitro bioactivity experiment was also carried out by incubating the SixHA in simulated body fluid (SBF) at 36.5 °C for 7 and 14 days. The obtained results revealed the substitution of SiO44- for some PO43- groups in the hydroxyapatite structure. The SixHA nucleated more apatite crystals on its surface and demonstrated some degradability during the periods of immersion in SBF. The characteristics of the SixHA imply that it could be used as a graft in bone restoration applications, thus signifying that sodium metasilicate could serve as an economic silica source for silicon-substituted hydroxyapatite production.
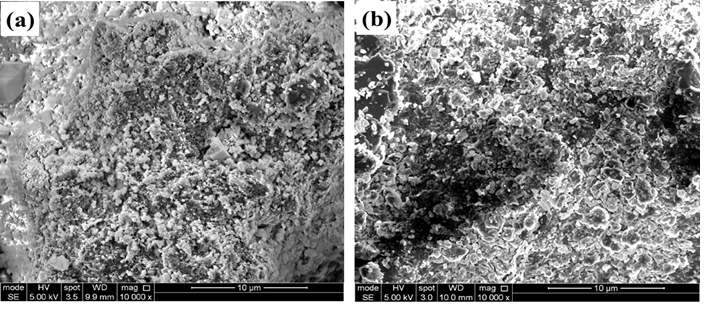
Published
How to Cite
Issue
Section
Copyright (c) 2024 Enobong R. Essien, Violette N. Atasie, Ngozi A. Adeleye, Luqman A. Adams

This work is licensed under a Creative Commons Attribution 4.0 International License.